Erweiterte Reichweite: die Faktendatei
Time to push network cabling beyond the limits
Like the roots of a growing tree, the enterprise network is branching out from the core and making its way into the farthest reaches of buildings and campuses as network managers try to stay one step ahead of the hyperconnected enterprise.
The internet of things (IoT) market is expected to have grown 18 percent in 2022, to 14.4 billion connected devices—and will include an estimated 27 billion devices by 2025.[i] The surge in connectivity is being fed by emerging and expanding edge-based technologies: advanced building automation and control, ubiquitous in-building wireless, campus-wide CCTV security and digital signage, smart manufacturing/distribution and more.
[i] State of IoT 2022; IOT Analytics, research report; 18. Mai 2022
The growth in edge-based connectivity presents a number of key challenges for enterprise network managers. Among them: how to extend the reach of their networks to deliver the required power and bandwidth beyond the traditional 100-meter distance limitation. Existing structured cabling architectures and standards can only go so far, and new extended-reach applications are continually emerging.
In this Enterprise Source fact file, we’ll dive deeper into the challenge of extended-reach power and data delivery. We’ll explore the various options and best practices for supporting connected devices at any distance from the telecom room (TR). Toward the end, we’ll show you how far a bit of out-of-the-box thinking can take you.
Before discussing how to solve the problem, let’s understand it a little better. Why exactly is there a 100 m limit?
The 100 m distance limitation as codified in the industry standards (namely, ANSI/TIA-568, ISO 11801 and other cabling standards for commercial buildings) is based on the electrical limitations of twisted-pair copper cabling. As the signal travels from one end of the cable to the other, its strength is affected by certain parameters, primarily insertion loss. The longer the cable, the greater the insertion loss. Based on these performance parameters, the industry standardized on the 100 m distance.
The distance limitation represents a worst case scenario for a given application and length when conducting a signal at the cable’s maximum frequency. It assumes a four-connector channel using a 90 m trunk and a 5 m patch cable at either end.
The limit was standardized in the 1990s and has stood the test of time, even as higher-frequency applications and new cable constructions have entered the market. In that time, network equipment vendors cost-optimized their transceivers based on the 100 m limit—further solidifying it as the accepted distance boundary.
On one hand, the 100 m channel limit has simplified the job of developing reliable performance specifications for new technologies extrapolating supportable distances for each new generation of cabling.
On the other hand, the distance barrier has created a new challenge for network designers. Enterprise networks are expanding faster than the budgets that are needed to implement and manage them. This is happening as available space for additional network components shrinks. As major trends such as Industry 4.0 and the onslaught of IoT/IIoT advance, network managers must be able to support more network-connected devices and systems—throughout buildings, across campuses and, especially, at the edge.
Möchten Sie offline lesen?
Laden Sie eine PDF-Version dieses Artikels herunter, um ihn später erneut zu lesen.
Bleiben Sie informiert!
Abonnieren Sie The Enterprise Source und erhalten Sie Updates, wenn neue Artikel veröffentlicht werden.
To reach connected devices located more than 100 m from the existing telecom room (TR), one solution is to simply add one or more TRs closer to the devices. But it’s not so simple.
From a space, cost and maintenance perspective, this approach is easily the most expensive—and almost impossible to justify for only one or two devices. An alternative is to deploy a wall-mounted cabinet with a switch and panel. While a “mini-TR” is less costly, space can be scarce, and there’s still the issue of running power to the cabinet.
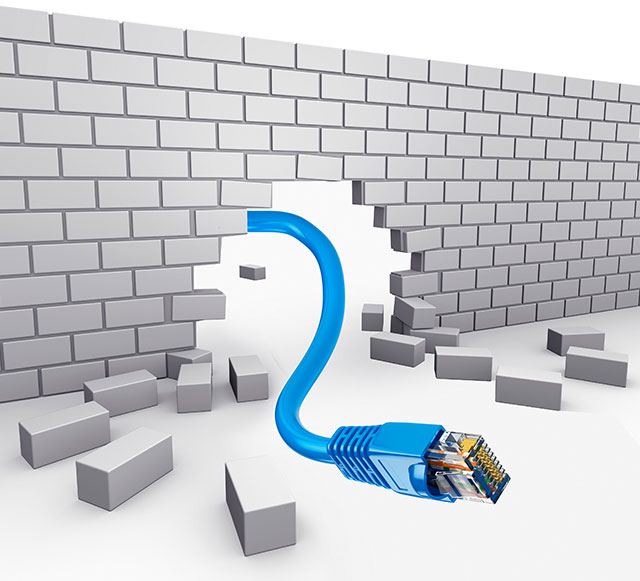
Pros
- Consistent architecture/media across the site
- Supports power over Ethernet (PoE)
- Supports up to 10G
Cons
- Not always possible
- Requires more space (and costs)
- Intermediate TR requires power
Another approach is to install a PoE extender in-line between the power source equipment and device. A PoE extender offers two major advantages. Each side of the link—from the power source equipment (PSE) to the extender and the extender to the device—can span 100 m, meaning network designers can essentially double the length of the channel. Some PoE extenders support daisy-chaining multiple units for spans of 500+ meters. It also provides a relatively inexpensive standards-based solution.
However, a PoE extender (aka “repeater”) is subject to the power and bandwidth limitations of the PoE technology and cable medium used. This means it can only serve a few devices. It also requires dedicated space and, without careful tracking and documentation of where extenders have been installed, management and troubleshooting can be difficult. While an extender allows networks to leverage the existing copper cabling, it requires local power, which can be difficult to access in some areas.
Pros
- Good solution for a few devices
- Inexpensive and standards based
- Uses existing copper plant
Cons
- Finding space can be difficult
- Hard to manage if all extenders aren’t carefully documented
- Power may be hard to access in some areas
- Difficult to troubleshoot
Yet another option is to deploy a system of hybrid cables containing copper conductors and fiber-optic cores that feed power and data to PoE extender devices. “Powered fiber,” as it is known, has the ability to extend the network coverage up to 3 kilometers, making it a good alternative for both in-building and campus-wide applications.
Powered fiber cabling solutions combine high-performance, low-latency fiber-optic data connectivity with a copper low-voltage direct current (dc) power connection. Dies ermöglicht den Anschluss einer beliebigen Anzahl von stromversorgten Remote-Geräten, ohne dass neue Leitungen verlegt werden müssen. With the powered fiber cable solution, the network gains access to a vast and growing ecosystem of applications, including:
- Notruftelefone
- HD-Überwachungskameras
- Digitale Beschilderung
- Wi-Fi access points (APs)
- Mikrostandorte
- Optisches LAN
- An array of low-voltage dc-powered devices
Powered fiber is widely used in situations where large areas need to be cost-effectively served by a single power and data network. These solutions, however, require more expensive fiber transmission equipment, as well as a Class 2 limited power source (LPS).
Pros
- Same architecture on a different media
- Future-proof solution for remote devices
- Requires no electrical skilled labor, since it is low voltage
Cons
- Requires a power supply in the telecom room and a PoE extender at the device end
- Maybe less cost-effective than other options
- Does not support 10G
Mehr erfahren: Faktendatei zu angetriebener glasfaser
A fourth option for delivering power and data to devices located over 100 m from the TR is to simply use a longer twisted-pair cabling link. While this method is not supported by the cabling standards, it is possible to use the application standards in conjunction with application testing to create a reliable link. Application standards help network designers gauge whether a specific application can run on a link segment, regardless of the cabling components used and the distance traversed.
For a more detailed analysis of this approach and the use of application standards and application testing, check out this article from Cabling Installation & Management.
Thoroughly vetted and correctly installed, the extended twisted-pair solution supports centralized management and efficient PoE delivery while reducing the number of link components and providing familiar RJ45 connectivity and installation.
Pros
- Easiest, least complex design
- Direct PoE from a PoE-enabled switch
- Familiar RJ45 connectivity and installation
- Familiar architecture
- Excellent data/power support for edge devices
- Easy to troubleshoot
- No additional points of failure
- Inexpensive
Cons
- Not supported by cabling standards
- Extra testing—in-lab and/or on-site
- Each new application must be re-vetted
- Not all high-bandwidth applications supported over extended distance
- Makes the design application-specific
Extending the traditional structured cabling network
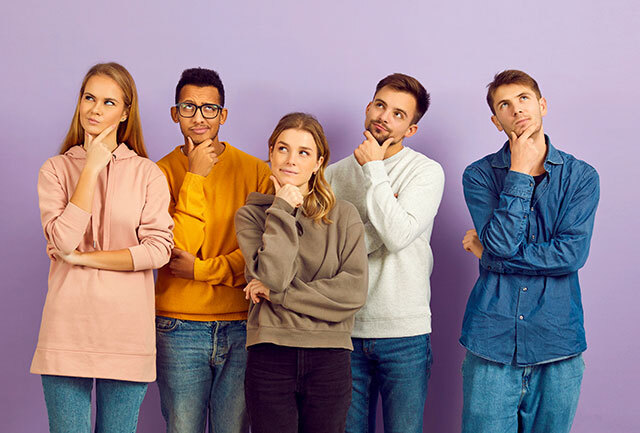
The four approaches described above will allow you to extend the 100 m reach of Ethernet. But, as you can see, each one has as many (if not more) arguments against it as for it. Of course, the balance of pro vs con for any approach may vary based on the specifics of the project.
Before we dismiss all four approaches as unfeasible, it may be useful to consider whether any of them could be “refined” to the point where the pros significantly outweigh the cons. (Spoiler alert: one of them can). As you read through the following arguments, keep in mind that, despite the challenges each one presents, there are specific use cases where they can and should be used. Our objective here is to see if there is one approach that can be used effectively in a majority of scenarios without significant drawbacks.
In the case of option 1, adding additional TRs, the cost, space and intermediate power requirements are something of a deal breaker. In many environments, there simply is not enough available room to locate the added equipment. Beyond the lack of space, the disruption of the normal workflow must also be considered.
Using POE extenders (option 2) gets us a bit closer. But here, the issue isn’t the time and cost of the installation, but the effective management of the network. Difficulty in troubleshooting issues is especially problematic. As service-level agreements for network uptime become more demanding, option 2 becomes less appealing. Let’s keep looking.
Option 3, powered fiber, addresses many of the installation and management issues created by the first two options. The major drawback here is one of capacity, as powered fiber currently maxes out at 10G. Just as we’d never have imagined needing gigabit service to endpoint devices 10 years ago, a few years from now, a 10G ceiling may seem low. Still, powered fiber could take us a few years down the road. Good but not great.
Extending the copper infrastructure (option 4) is interesting. On the plus side, it is certainly the easiest and most cost-efficient solution to implement, provides a familiar platform and RJ45 connectivity, and offers good management. The drawback here is performance verification and lack of a standards-support roadmap. If we could mitigate these last two issues, we may just have a winner—without having to reinvent the wheel. Hmmm, interesting. But before leaping ahead to a potential solution, let’s take a step back and dive a bit deeper into the 100 m limitation from a slightly different perspective.
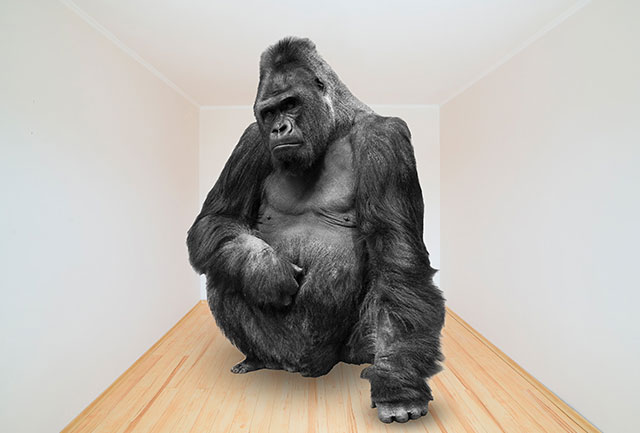
There are several considerations to consider before making a decision to design structured cabling with extended reach for some edge devices. No more daunting or important than solving the issue of the 100 m distance limitation.
We’ve already touched on this issue above, but the context there was primarily performance-related (frequency, insertion loss, resistance, temperature, propagation, etc.). What about the effects of the 100 m barrier on cable and connectivity providers?
We know the velocity at which a signal travels down individual pairs in a four-pair cable impacts the cable’s ability to support extended distances. We’ve also mentioned that cabling standards are designed for a worst-case scenario and/or minimally compliant components. In response, reputable cabling and connectivity vendors build in “additional headroom” by ensuring their products exceed the standards. For lower-bandwidth devices—such as access control devices like parking lot entrance gates, emergency call boxes and security cameras—the extra headroom isn’t necessary.
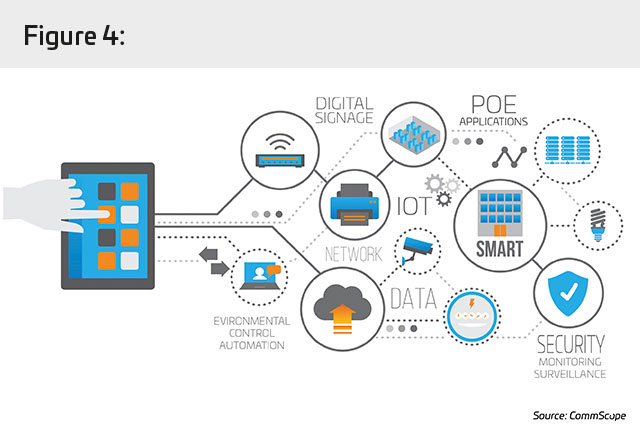
Other applications do require the channel’s maximum available bandwidth. Therein lies the challenge: today’s convergent enterprise networks must support a mix of low-, medium- and high-bandwidth applications as well as those needing low, medium, and high power.
But remember, our primary goal here is extending the distance, which is affected by bandwidth and power requirements but not defined by them. In fact, in a converged network, there are many low- or medium-rate applications that benefit substantially from extended-reach cabling:
- Building environmental control automation
- Security monitoring and surveillance
- Digital signage systems
- Enhanced PoE applications
- IoT devices and sensors
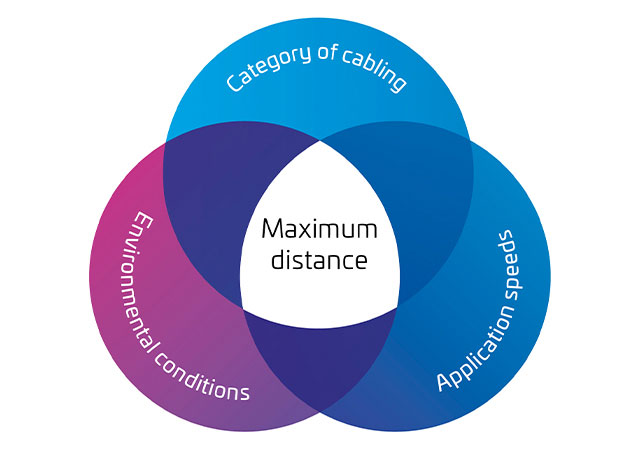
The maximum distance will depend on:
- Category of cabling used
- Application speeds to be supported
- Environmental conditions such as operating temperatures
For example, a PoE+ (PoE type 2) application can support up to 30 watts of power over 150 m or more. The maximum distance depends on the speed required and type of device supported:
- Maximum horizontal distance at 10 Mbps: 185 m
- Maximum horizontal distance at 100 Mbps: 150 m
- Video surveillance (1080p HD or 4K UHD): 150 m
All numbers above from Application Guide: Utility Grade Infrastructure (UTG)
Utility grade infrastructure
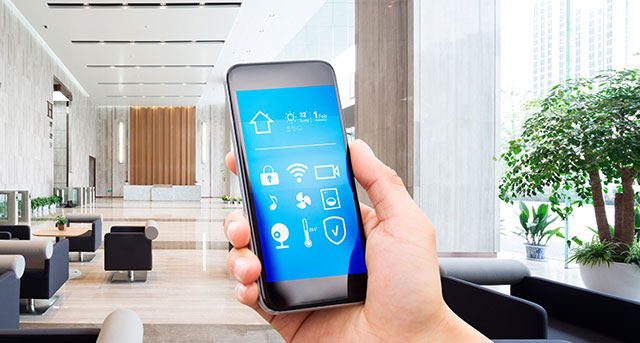
To this point, we’ve discussed the challenges of extending the network’s reach to support powered and connected devices beyond the 100 m limit defined in the cabling standards. Yes, there are “workarounds” like PoE extenders and powered fiber, which can support extended distances, but at a cost that makes them less than optimal. Now, we’d like to propose a better solution—one that enables building owners and their network managers to more efficiently address the issue of extended reach and more.
First, a bit of context.
Led by the surging demand for seamlessly connected building automation and controls, and convergence of IT and OT applications, data communication is now viewed as the fourth utility, as critical to an organization’s operational future as electricity, water and gas.
The challenge lies in creating an integrated suite of connectivity solutions without adding layers of complexity and cost. Success starts with a superior infrastructure that’s flexible enough to scale and integrate tomorrow’s technologies while managing multiple systems that require advanced power distribution, more bandwidth, and seamless connectivity.
UTG is a complete technology platform/assurance program/design approach. It unlocks a smart building’s full potential by redefining the infrastructure layer to support building subsystems, technologies and applications.
The UTG infrastructure platform features:
- Utility-ready structured cabling and connectivity from CommScope
- Advanced, tested power delivery
- UL verification of all performance and application claims
As a single converged infrastructure platform, UTG solutions bridge the gap between information technology (IT) and operational technology (OT). Now, building owners, operators and managers can navigate seamlessly between a broad range of technologies while cost-effectively improving network reliability and performance.
Benefits of a UTG infrastructure solution
- Supports IT/OT network convergence
- Advanced power distribution
- UL certified for many extended-reach applications*
- Provides optimal bandwidth for applications, systems and devices
- Improves scalability and design flexibility
- Reduces strain on infrastructure
- Extended cabling lifespan is more sustainable
- Meets productivity and efficiency demands
- Improves network reliability, reduces costs
- Enhances user experience
- Infrastructure management and security
*Based on application speed
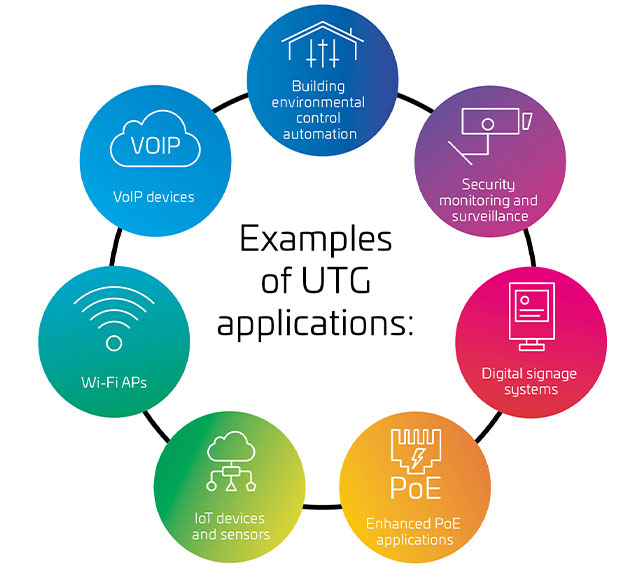
UTG infrastructure enables a range of existing and next-generation network capabilities, from standardized applications such as connecting Wi-Fi access points (APs) or IP phones, to those whose needs go beyond the standards, like extended distance, power, and cybersecurity.
Examples of UTG applications include:
- Building environmental control automation
- Security monitoring and surveillance
- Digital signage systems
- Enhanced PoE applications
- IoT devices and sensors
- Wi-Fi APs
- VoIP devices
Managing multiple systems on one robust platform accelerates efficiencies, increases business intelligence, strengthens cybersecurity, enhances productivity, optimizes performance and reduces operational costs.
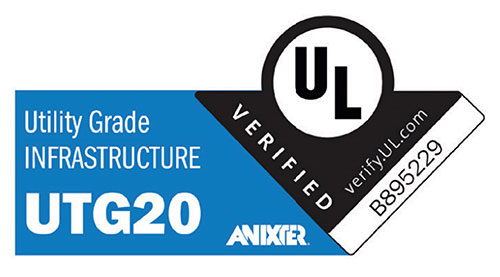
It will take time for the standards bodies to catch up to the extended-reach performance of the UTG infrastructure platform. Therefore, CommScope has worked alongside Anixter, UL and other third-party technology experts to validate all performance claims. These efforts have resulted in UTG-specific performance standards and UTG-rated infrastructure cabling solutions. Together, they support diverse applications, systems and devices on a common network.
All performance claims and UTG ratings are independently tested and verified by UL laboratories for optimal application assurance and design on a single building platform. The results are based on real-world, definitive application testing and UTG-exclusive testing protocols. Based on UL’s testing, CommScope’s UTG solutions outperform non-UTG cabling.
Customers deploying UTG infrastructure solutions enjoy a similar level of application support available through CommScope’s SYSTIMAX Application Assurance program. This support provides the design capabilities and performance helps building owners and enterprises need to migrate with confidence. In fact, the UTG program is highly complementary with the SYSTIMAX Application Assurance program, and we believe we can build on it to extend beyond what we currently support.
CommScope continues to focus on innovation and continual improvement, investing almost $200 million in R&D, including investments in our SYSTIMAX solutions. These investments, combined with a UL verification program to validate emerging and enhanced technologies, give our customers the satisfaction of knowing their CommScope products and solutions are indeed best-in-class.
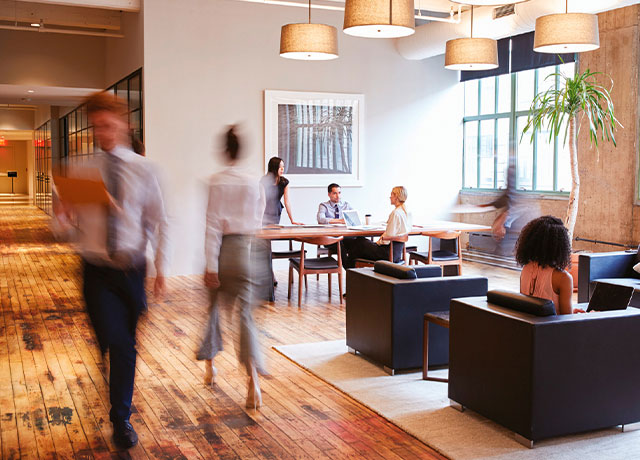
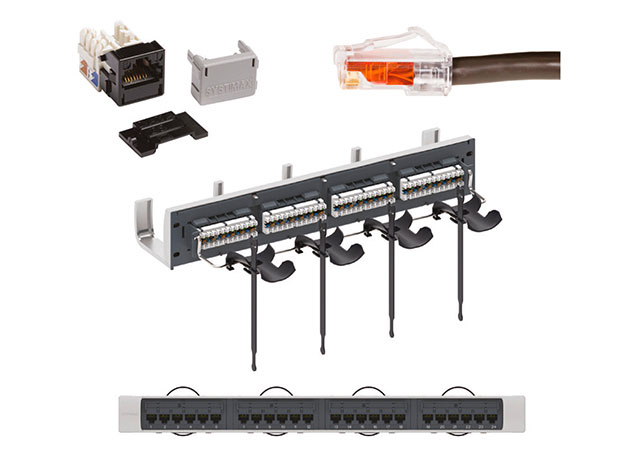
For more information on CommScope’s UTG infrastructure portfolio:
Extended-reach testing
To enable extended coverage and enhanced flexible network design, CommScope has conducted extensive testing to ensure reliable coverage beyond the traditional 100 m requirements. Combining carefully selected products from our leading SYSTIMAX® and RUCKUS® portfolios, the testing demonstrates flawless network functionality—offering customers the ability to leverage a closed PoE-based ecosystem that extends the length over which traditional switch/AP combinations will support Wi-Fi networks up to 180 m or 600 feet.
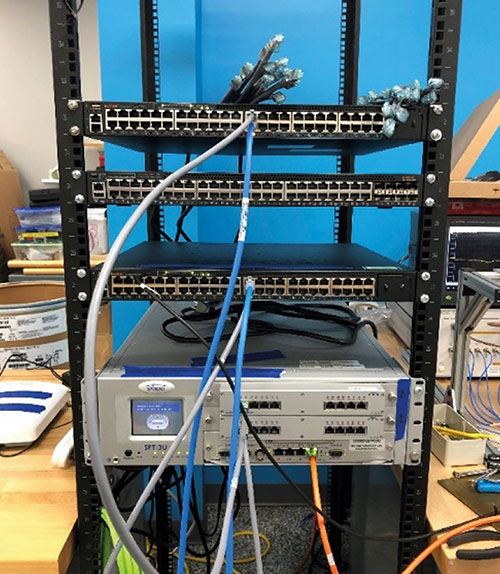
Equipment used
RUCKUS ICX7650-48ZP multi-gigabit PoE switch (ports 25 through 48):
100 MbE/1 GbE/ 2.5 GbE/5 GbE/10 GbE POH capable
GigaSPEED® Category 6A cable channel:
configured with up to four connections using GigaSPEED X10D® 2091B cable and 360GS10E cords
RUCKUS R850 AP:
Wi-Fi 6 PoE enabled, supports 5GBASE-T and 2.5GBASE-T applications
- 5GBASE-T applications are supported when the R750 AP is connected to ports 25 to 48 of the ICX7650-48ZP using up to 180 m of GigaSPEED X10D cabling
- 5GBASE-T applications are supported when the R850 access point is connected to ports 25 to 48 of the ICX7650-48ZP using up to 180 m of GigaSPEED X10D cabling
- 5GBASE-T applications are supported when the R850 access point is connected to ports 25 to 48 of the ICX7650-48ZP using up to 125 m of GigaSPEED X10D cabling
It is important to emphasize that these results were achieved using a “closed-loop” channel in which all equipment, cabling and connectors were engineered and manufactured by CommScope. While similar results using other manufacturers’ components may be possible, channel performance over extended distances cannot be guaranteed using non-CommScope components.
For a detailed analysis of the extended reach testing, results and limiting parameters, download the Extended reach installation guidelines.
Cabling parameters that can affect reach and performance
Having outlined the issues of extended-reach performance, and proposing several existing solutions (and one new and innovative solution), we now turn our attention to understanding how to get the most out of your chosen strategy.
There are a number of characteristics, both electrical and physical, that impact a cable’s ability to adequately sustain signal performance over the length of the channel.
Electrical parameters include insertion loss, resistance unbalance, propagation delay and mismatched impedance. On the physical side, variables such as including the conductor’s diameter and cordage design also affect reach and performance, as does the category of cable used.
Insertion loss (aka “attenuation”) is the reduction in signal strength suffered by an electrical signal as it propagates along a transmission medium, and it is present in all media types. It determines the maximum distance over which a transmitted signal can be resolved and, therefore, the maximum distance two devices can be separated while maintaining communication.
Insertion loss is usually expressed in decibels per unit length (e.g., dB/1,000 feet) and is a measure of how much a signal is weakened or reduced in amplitude as it travels down a cable. It also increases as the cable’s operating temperature increases. In a copper wire, insertion loss is caused by two main factors:
Copper loss is related to the thickness of copper used in the construction of the cable. Increasing the copper would inflate the cost of the cable and be unnecessary for the vast majority of installations that are less than 100 m in length. Increasing the conductor size also impacts the ability of the conductor to mate properly with connectivity in the form of jacks and/or plugs. Hence, dramatic increases in attenuation would be achievable only with the adoption of a new connector—something most users do not want.
Dielectric loss (aka “dissipation”) is related to the electrically lossy properties of the insulation and jacketing materials used to construct the cable. The choice of insulating and jacketing material impacts the insertion loss of the cable but also determines how the cable performs during important safety tests such as those intended to characterize flammability and smoke release. Often, tradeoffs between the electrical and safety performance of a cable dictate the types of materials employed. This is further complicated by the existence of regional safety standards such as those for plenum rated and low-smoke-zero-halogen rated environments.
It’s important to note that there are other factors that can influence insertion loss, such as resistance and heat. For example, the maximum allowed insertion loss for Category 6A (at 500 MHz) is 42.8 dB, compared to Category 6 (at 250 MHz), which is 31.1 dB. This is also why links involving smaller-gauge wires with more resistance, and where the ambient temperature is above 20°C (68°F), can require length de-rating. The higher the insertion loss, the shorter the reach must be for a signal at the far end of the link to be understandable. This is a key reason why insertion loss has such an important impact on the supported distance.
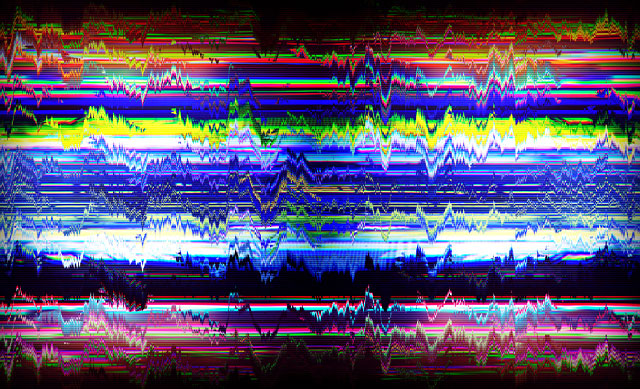
SNR is directly related to insertion loss. Measured in dB, it expresses the ratio of a link’s signal power compared to the noise power for a given frequency range. The higher the ratio, the better the signal quality. SNR and insertion loss are inversely related—the lower the insertion loss, the higher the SNR. Just as insertion loss increases with distance, so too SNR is affected by cabling length. Should the SNR drop to unacceptable levels, either the transmission or cable length must be reduced to support the application.
Conductor size will also affect maximum cable length. The amount of resistance is inversely proportional to the thickness of the gauge (due to the skin effect). Therefore, larger gauge wires will exhibit better insertion loss. In some cases, using a cable with 22 or 23 AWG conductors instead of smaller 24 AWG conductors makes more sense for extended-reach applications. While this makes sense for some isolated applications, it would be impractical to equip an entire building with 22 AWG cable just in case some of the runs are longer than the standard 100 m. 24 AWG and it’s cost effective.
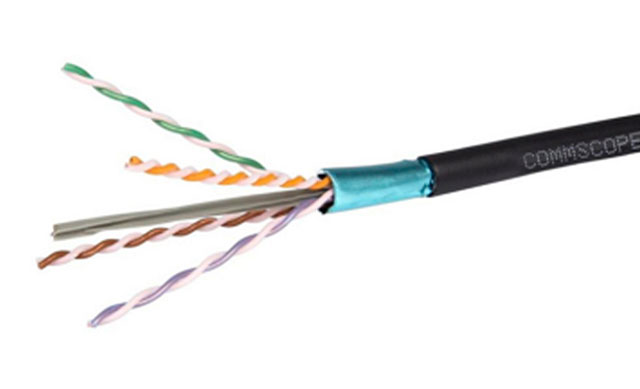
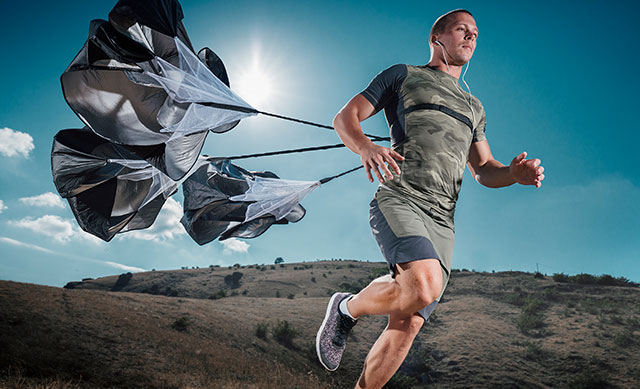
Electrical resistance is the conductor’s ability to resist the flow of electrons. Direct current (dc) loop resistance, measuring the resistance of two connected dc conductors, indicates how efficiently the loop delivers power in a PoE application. ANSI/TIA-568.2-D and TIA TSB-184A D3.0 specify that dc loop resistance (resistance unbalance) for Category 3/5e/6/6A shall not exceed 25 ohms; exceeding the dc loop resistance limit creates heat within the cables, which increases insertion loss and reduces the acceptable link span. To ensure safe operation over extended distances, many extended-reach cables include additional resistance headroom to account for the unbalance.
The first Cat 6A cables and patch cords were bulky and rigid. Newer patch cords employing smaller conductors (usually stranded and thinner than 24 AWG) are appreciated by customers given their flexibility and less volume they fill in cord organizers. However, smaller conductor cords, no matter how short they are, have an impact on the total insertion loss and may also imply some length de-rating. There is a balance to be found between comfort and performance!
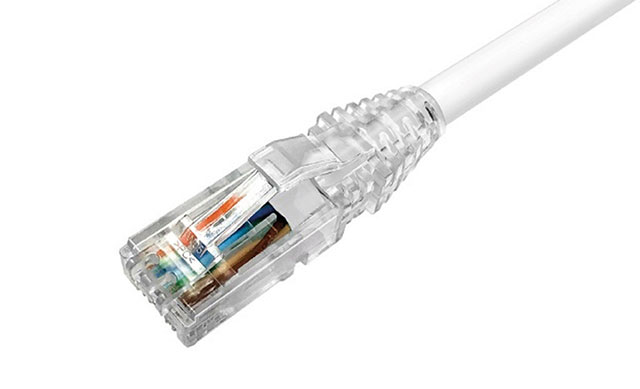
Propagation delay is the time needed for a signal initiated at one end of the channel to be received at the opposite end. In twisted-pair cabling, this time interval is impacted by the cable’s length and operating frequency, previously discussed, as well as a third factor: nominal velocity of propagation (NVP). NVP is a relative measure of the signal’s speed compared to the speed of light in a vacuum (c). Typical structured wiring cables exhibit an NVP of 0.6 c to 0.9 c, meaning the signal travels at 60 to 90 percent the speed of light in a vacuum.
A cable’s maximum NVP is dictated, in part, by physical characteristics such as the number and tightness of twists in a pair of strands. As the number of twisted pairs in a cable bundle increases, the chances of disparities in the twists among pairs also increase. The difference in propagation speeds between the fastest and slowest pairs is known as the “propagation delay skew.” As propagation delay skew grows, the network equipment has more difficulty reading the signal. Extended cable lengths can exacerbate this issue.
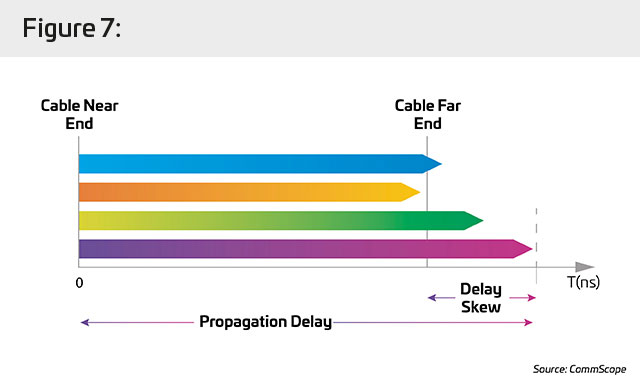
Conductor size will also affect maximum cable length. The amount of resistance is inversely proportional to the thickness of the gauge (due to the skin effect). Therefore, larger gauge wires will exhibit better insertion loss. In some cases, using a cable with 22 or 23 AWG conductors instead of smaller 24 AWG conductors makes more sense for extended-reach applications. While this makes sense for some isolated applications, it would be impractical to equip an entire building with 22 AWG cable just in case some of the runs are longer than the standard 100 m. 24 AWG and it’s cost effective.
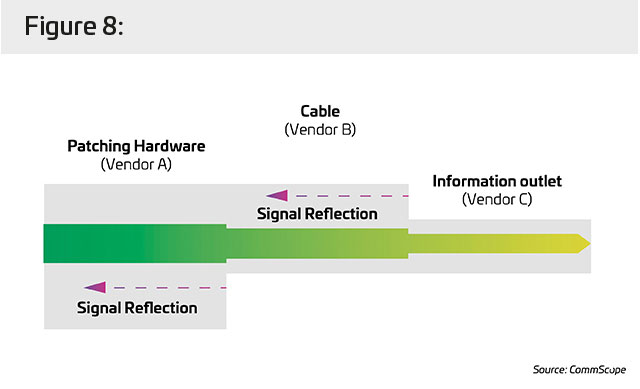
When we compare the different categories’ performances in light of the aforementioned parameters, it’s easy to understand the advantage of Cat 6A when we need to go further than the standard 100 m:
- At 100 MHz, Category 5e has an insertion loss maximum of 24 dB, while Category 6 is at 21.3 and Category 6A is at 20.9 (per TIA channel specifications). Remember: The lower the dB for insertion loss, the better.
- Common 6A cable and cordage gauge is larger (thicker) than for other categories, which improves insertion loss, resistance and SNR parameters.
- Cable heating is lower for 6A when transmitting the same current and wattage for PoE purposes, which makes possible larger cable bundles.
- As previously mentioned, first-class 6A solutions are carefully tuned up to provide lower signal reflection.
- All of the above advantages, combined with the inherently superior performance for alien crosstalk, make Cat 6A the perfect choice.
Fazit
The changes in how organizations deploy, use and manage data are, in a word, transformative. Increasing use of augmented reality, IoT, and building automation/control are leading to increased workforce productivity, collaborations and safety, and buildings that are more efficient and sustainable. For network managers, however, designing networks that can support and sustain these new capabilities is a significant challenge.
While networks—including IT, OT, power and data—are converging to become more efficient, the number of connected devices and systems is exploding and moving to the network edge, closer to where data is being created and consumed. Supporting these changes means rethinking the role, design and capabilities of the structured cabling infrastructure, something CommScope anticipated years ago.
As an industry leader and innovator, we began working long ago with UL, Anixter and other network engineering specialists to develop an evolutionary unified infrastructure platform: UTG infrastructure. Now, customers can confidently extend the reach of their structured cabling networks to support tomorrow’s next-generation connected devices and systems.
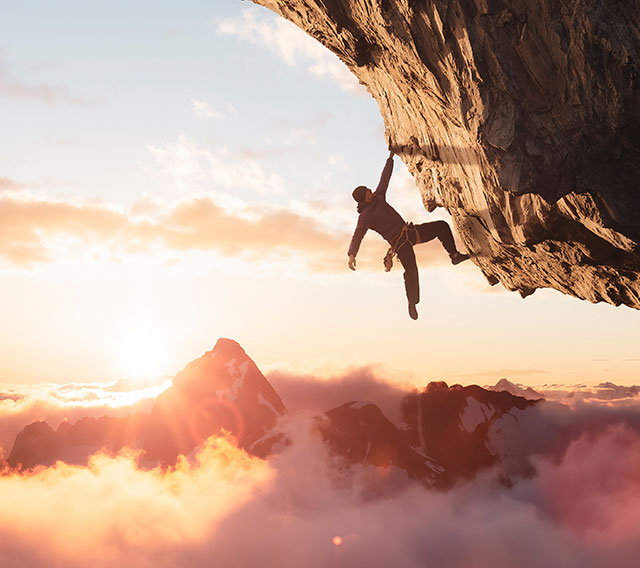
Wie man an einem 100 Meter langen Gorilla, der Ihr Netzwerk als Geisel genommen hat, vorbeikommt
Innovative Strategien, um die Reichweite Ihres Netzwerks in einer schwierigen Umgebung zu vergrößern